Reading time: 18 minutes
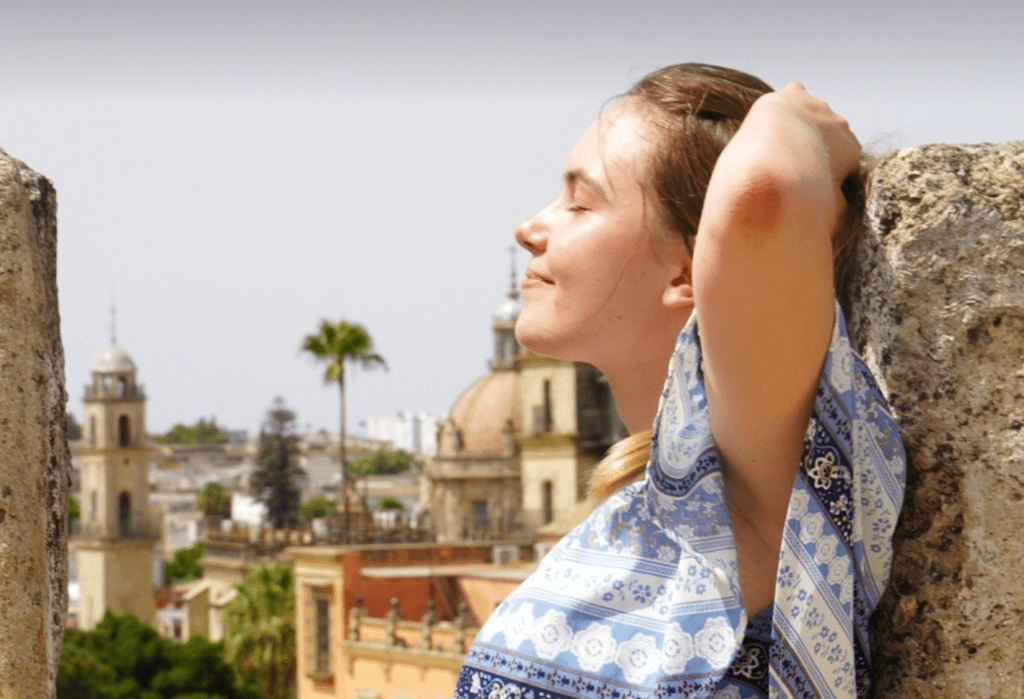
THINGS TO KNOW Summer is over, the sun is lower above the horizon, and the sun’s radiation becomes weaker overall. The probability of getting a sunburn also decreases significantly. But what is the actual reason for this? Why can’t you get sunburn from visible light and where does it come from instead? In a nutshell, the physical answer is: It has to do with the radiation spectrum of our sun and the size of energy packets. And with that we are already in the middle of quantum physics.
The interaction of solar radiation with the skin is complex and can hardly be considered sufficiently in an article of this scope. But the physical mechanism behind a sunburn can be modeled in a comparatively simple way: Light hits the skin in energy packets called light quanta. Depending on the size of the energy packets, this can lead to damage to the sensitive DNA and RNA molecules in the skin cells. UV-B radiation from our sun is particularly dangerous.
For a long time it was not clear that light consists of energy quanta. Until the end of the 19th century, it was still imagined as a wave. The wave-like propagation of light (similar to that of sound waves) was already known in 1802 by small experiments of Thomas Young and so it was assumed that the energy transfer also takes place “wave-like”, i.e. continuously and depending on the intensity of the light radiation. That the latter assumption could not be true was shown by the outer photoelectric effect discovered in 1888 by the German physicist Wilhelm Hallwachs.
The external photoelectric effect and the light quantum hypothesis
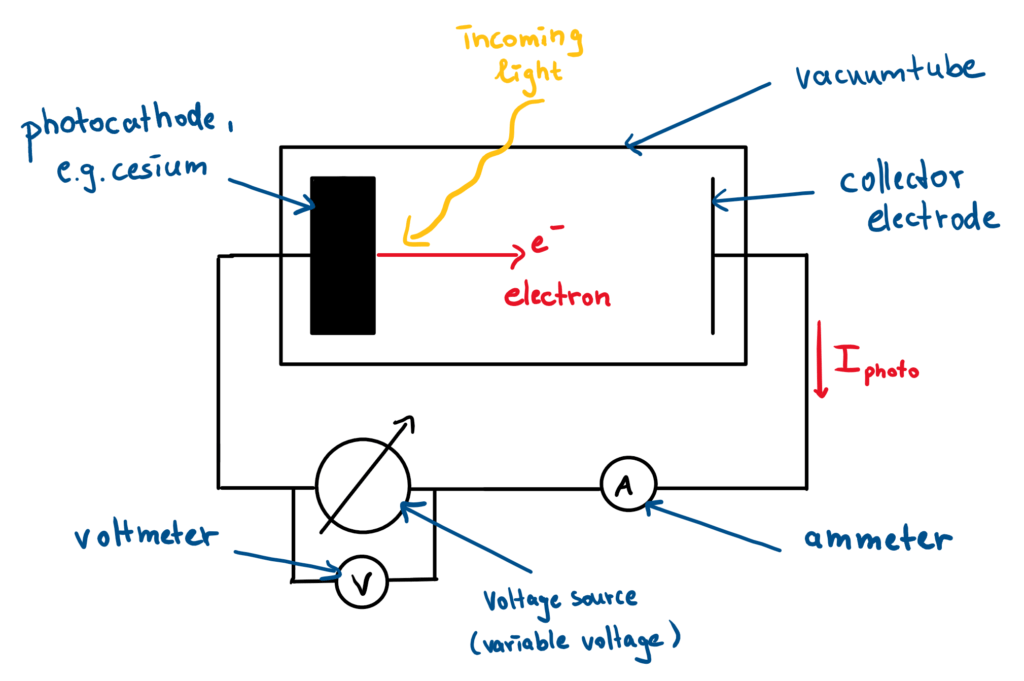
A simple experimental sketch makes the effect somewhat clearer (Fig. 1). When light falls on a photocathode, which are usually alkali metals like cesium, this light transfers energy to the electrons sitting in the metal surface. If the transferred energy is large enough, these elementary particles can be released from the material and fly with a certain kinetic energy to the collector electrode (anode).
If the photocathode and collector electrode are connected via an electric circuit, a photocurrent is generated. This is because the small, electrically negatively charged electrons now move (and their charge) in the direction of the increasingly positively charged cathode. The resulting current can be easily determined using an ammeter. To determine how much kinetic energy was added to the electrons when they were triggered, a variable voltage source can be added to the circuit (measured with a voltmeter).
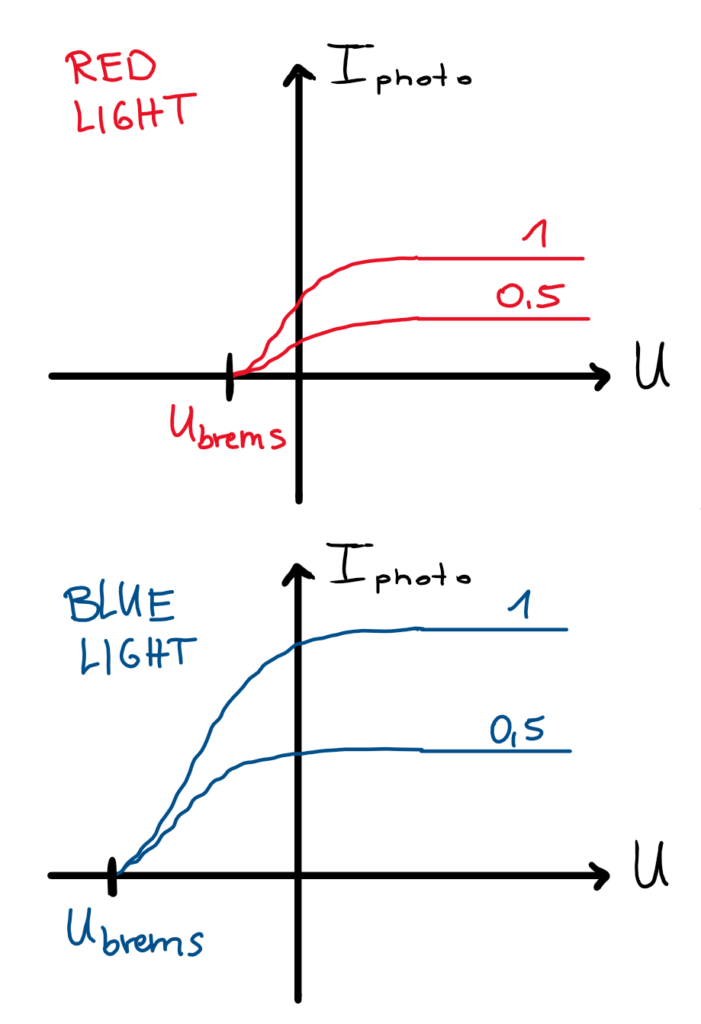
If the generated additional voltage points in the opposite direction to that from the photocurrent, the electrons are slowed down, because now part of their kinetic energy is used up for “fighting against the countervoltage”. If the countervoltage is large enough, the maximum kinetic energy can be calculated from the formula for the acceleration voltage Ekin,max = e*Ubrems, if e is the charge of the electrons and Ubrems is exactly the countervoltage at which the photocurrent drops to zero.
If the externally applied voltage U is plotted against the measured photocurrent for different intensities and light colors, the picture on the right is obtained (Fig. 2, sketch). It can be seen that a larger photocurrent can be measured at higher light intensity (1) than at half intensity (0.5), so more electrons are triggered. Furthermore, for each intensity, a certain saturation can be seen above a certain positive accelerating voltage (voltage gives electrons more energy). This means that – even if the electrons are still accelerated – only a certain number of electrons are released from the surface and the photocurrent cannot rise above a value characteristic for the respective curve.
In the range of the negative accelerating voltage (counter voltage), on the other hand, one recognizes a common feature of the curves: The braking voltage, from which one can read the maximum kinetic energy of the electrons, assumes the same value independent of the light intensity. This value seems to depend only on the color of the light used. For blue light it has a larger magnitude than for red light. This refutes the thesis that light energy is transmitted in waves only as a function of light intensity. Instead, another criterion comes to the fore: the color and thus the frequency of the light.
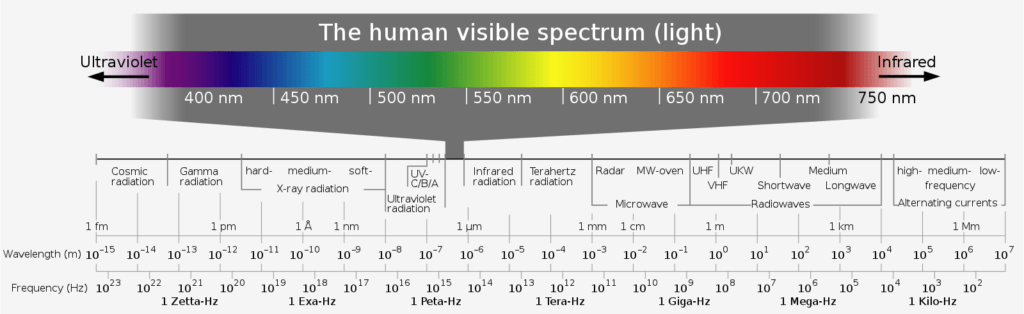
Today we know that visible light consists of electromagnetic waves. It is characterized by its wavelength and ranges from about 400 nanometers (purple) to 700 nanometers wavelength (red), thus covering a frequency range from about 7.5*1014 Hz (purple) to 4.3*1014 Hz (red) – after all, frequency = speed of light/wavelength. However, the spectrum of all electromagnetic waves extends much further: from gamma radiation to X-rays and ultraviolet radiation (very energetic, large frequency) to infrared radiation and microwaves (low energy, small frequency).
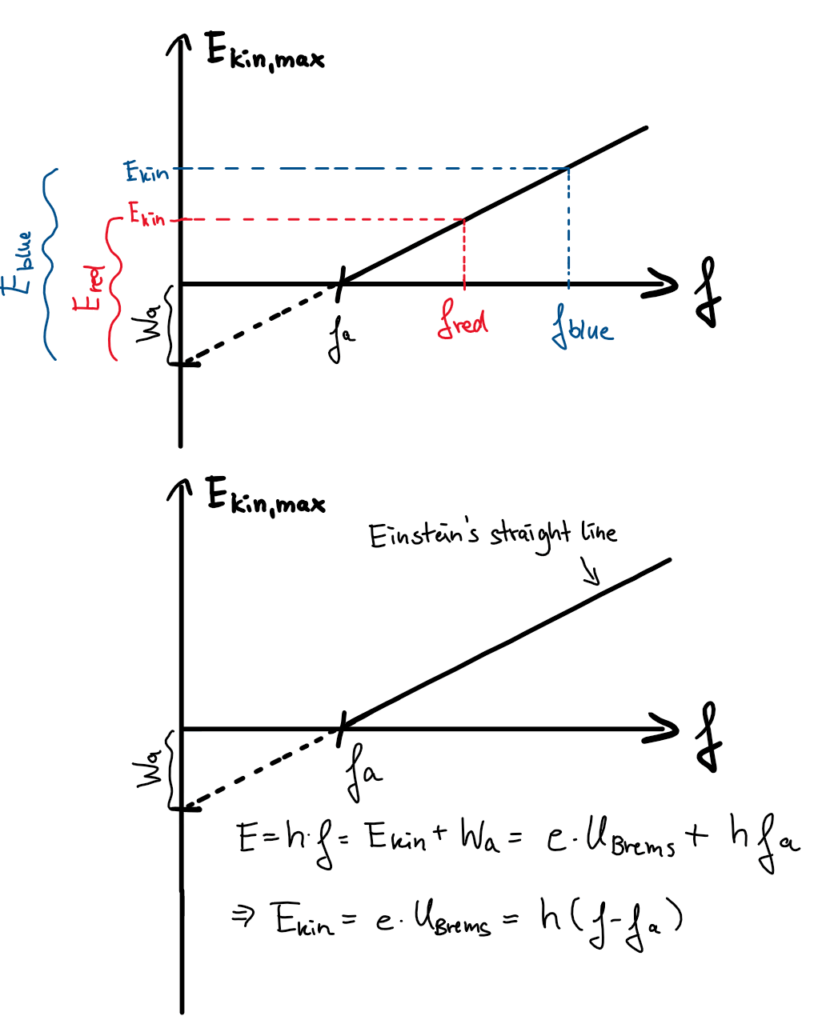
Blue light has a higher frequency than red light. Moreover, the energy transmitted by blue light appears to be greater than that transmitted by red light. This suggests that the greater the frequency of the light, the greater the energy transmitted.
To investigate this claim further, one can plot the maximum transmitted kinetic energy as a function of light frequency. This results in a diagram similar to those in Figure 4. In fact, the transmitted kinetic energy increases with frequency: The result is the so-called Einstein straight line. However, this line intersects the x-axis at a point which corresponds to the cutoff frequency fa. If the irradiated light has a frequency f < fa, no electrons can be triggered regardless of the light intensity. How can this observation be explained?
Einstein provided the answer in 1905 with his light quantum hypothesis and was even awarded the Nobel Prize for it in 1921. He described light as energy packets, i.e. light quanta or also called photons. Each photon has a characteristic energy of E = h*f, where f is the frequency of the light in question and h is Planck’s quantum of action (h = 6.6*10-34 Js). When light hits an electron, exactly in photon can transfer its energy to the electron. Only if the energy packet is larger than the so-called work function Wa= h*fa, then the release takes place and the remaining energy forms the kinetic energy of the electron.
Sunburned – the radiation of our sun
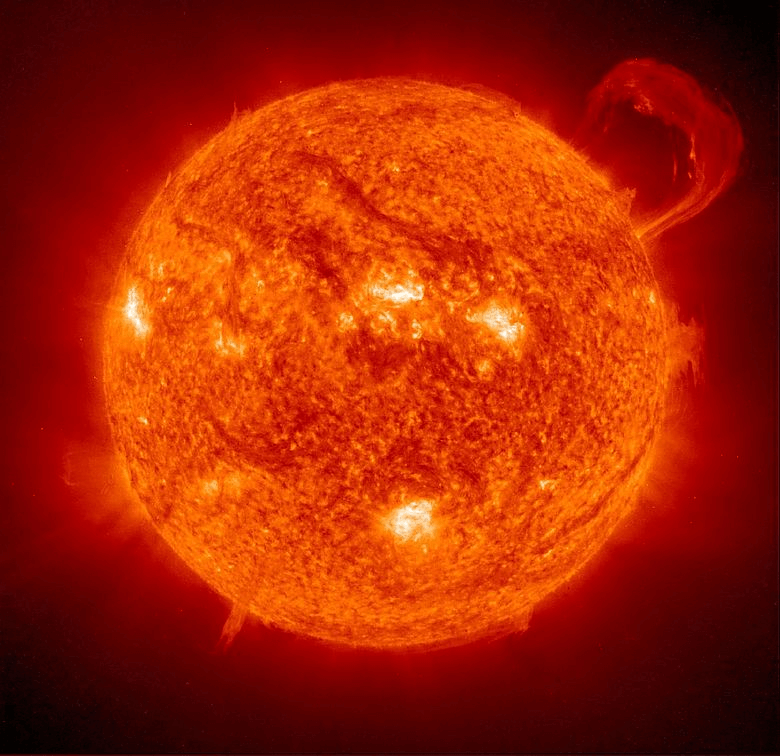
Source:
https://commons.wikimedia.org/wiki/File:Csm_
WieSo_Verlust_Masse_Sonne_f6d924ecef.jpg
But what does this mean for us? Well, it has on the one hand to the consequence, what I simply asserted at the very beginning of the article: That visible light is not enough to cause sunburn. Much more dangerous is the so-called ultraviolet radiation. To understand this better, it is worthwhile to examine the solar spectrum more closely (Fig. 6). The sun is a black body. Its surface is very hot at 5900 K (about 10160 °F) and emits optical radiation that has its maximum intensity in the visible light range, but ultraviolet and infrared radiation also strike the earth.
Based on the light quantum hypothesis, we know that infrared radiation, which we perceive as heat, has a smaller frequency than visible light. It therefore hits the earth in the form of smaller energy packets and cannot cause any damage to our skin. It is the same with visible light. These photons have a slightly higher frequency, but this is still not enough to really damage our skin. That is why we cannot get sunburn from visible light.
However, UV-A radiation and UV-B radiation also hit the earth. These are much more energetic and can cause both short-term and long-term damage to the body. The skin ages prematurely, the immune system is weakened, eyes are damaged and, last but not least, the risk of skin cancer increases. Sunburn itself occurs when the high-frequency UV-B photons attack the skin’s DNA molecules and RNA molecules, leading to a kind of inflammation of the skin in a chain reaction.
Example: You cannot get sunburn in a car because the windshield filters out both UV-A and UV-B radiation from the sun. Nevertheless, you should protect your skin, especially during long car trips in summer. This is because the side windows still allow some UV-A radiation to pass through, and even if this does not directly cause sunburn, the energy packets are quite sufficient to cause long-term damage to the skin.
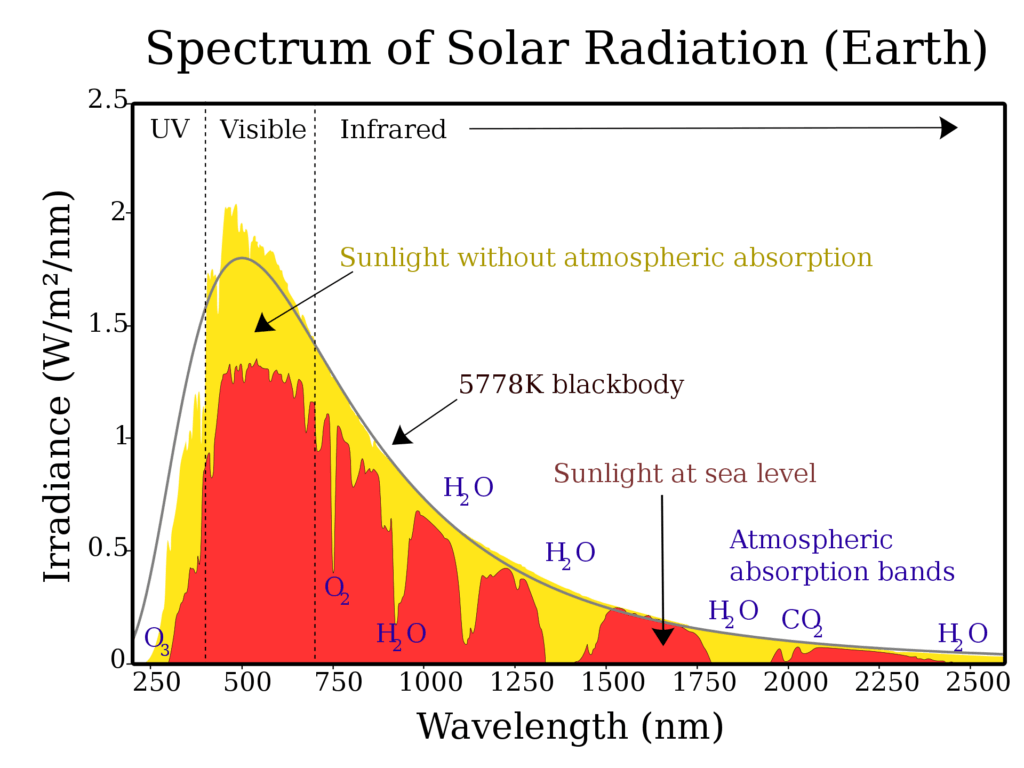
Source: https://en.wikipedia.org/wiki/Solar_irradiance#/
media/File:Solar_spectrum_en.svg
The exact interaction processes between skin and electromagnetic radiation have not yet been clarified. However, it is known that in addition to the positive effect of the formation of vitamin D3 by UV radiation, the negative effects (especially of the even stronger UV-B radiation) should not be underestimated. The more UV radiation hits the skin – the greater the intensity of the radiation – the greater the damage caused.
Now it is the case that not all of the radiation emitted by the black body “sun” reaches the earth. A large part is absorbed by the earth’s atmosphere (see Fig.6). Thus, the very energetic UV-C radiation does not even reach the earth’s surface, and only about ten percent of the UV-B light emitted by the sun reaches the human skin. In addition, the intensity of the radiation is strongly dependent on the current weather and position of the sun.
The UV index indicates the daily peak value of harmful UV radiation expected at a specific location. It is calculated according to the following criteria, among others:
- The flatter the sun is in the sky, the smaller the radiation intensity, because then the same radiation is distributed over a larger area. In addition, the longer the path through the atmosphere, the greater the energy losses. Thus, the damage caused by UV radiation is greatest at the equator/summer/at midday.
- The clearer the air and the higher the altitude, the deeper radiation penetrates to us.
- Heavy cloud cover can reduce the intensity of UV radiation.
- Snow, sand, water and asphalt reflect UV radiation.
The most important facts in brief
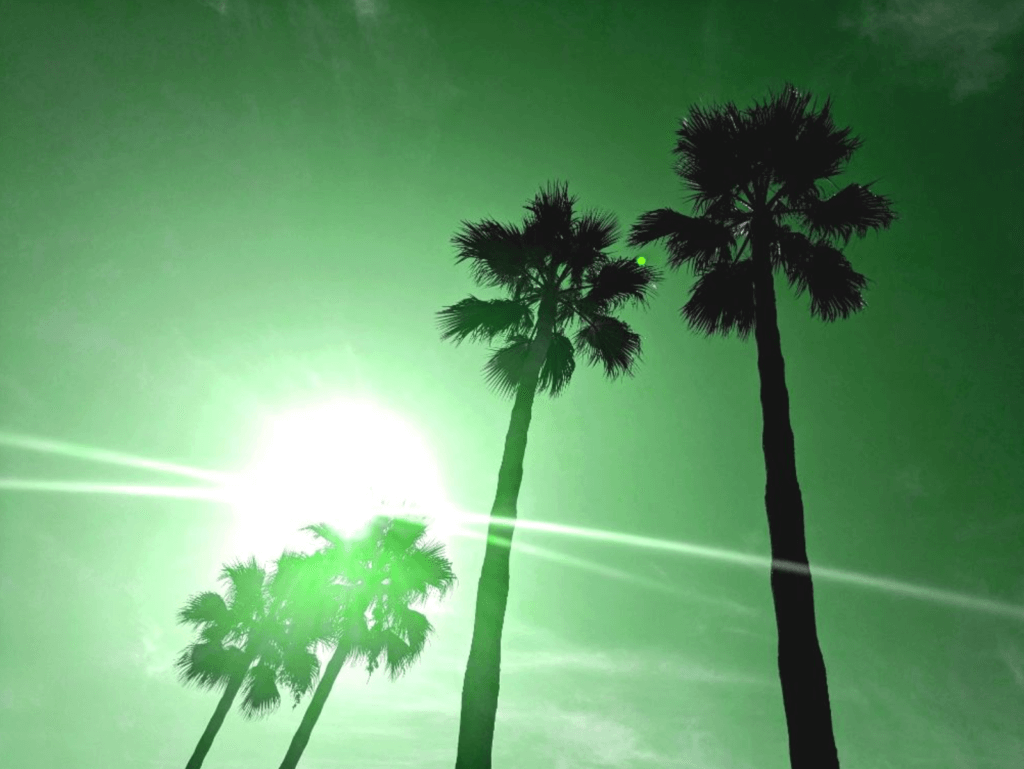
The external photoelectric effect shows that electrons can be released from the surface of metals by exposure to light. The number of electrons increases with the intensity of the light. However, electrons are not released at all until the frequency of the light rays exceeds a certain limit. Einstein explains the effect by the fact that light consists of energy packets with the energy E=h*f. A photon transfers thereby its energy to an electron and brings up thus first the necessary exit work, the remaining part of the energy goes into the kinetic energy of the electrons.
Electromagnetic radiation in the infrared, visible and ultraviolet range hits the earth from the sun. Only the energy packets of the ultraviolet rays are sufficient to cause lasting damage to the skin and can cause sunburn. The intensity of the light radiation is decisive for the strength of the damage and depends on the wavelength of the light, the current weather conditions and the position of the sun. So, depending on the location and time, it is definitely important to protect your skin from harmful UV radiation.
A note on my part: The fact that light sometimes behaves like a wave (wave-like propagation and interference effects) and sometimes like a particle (energy or momentum transfer) led, among other things, to the development of wave-particle duality. Today it is assumed that light is neither a wave nor consists of particles. Light quanta are considered to be simply quantum objects. Electrons and other microobjects also exhibit wave properties. Their properties, which depend among other things on whether the particles are observed, are described by quantum physics. "Anyone who thinks he has understood quantum theory has not understood it" - Richard Feynman. Admittedly, this statement seems outdated nowadays. In the meantime, quantum mechanics has indeed been understood to a large extent. Today, many previously unimaginable phenomena can not only be understood theoretically, but even detected, such as the entanglement of two particles that are located in completely different places. But even now, after two years of studying physics, most quantum mechanical phenomena still completely elude my imagination. No one knows one hundred percent whether our current physical theories are actually true. But even if only a small part of the theories should be true, it is incomprehensible to me what an incredible world we live in.
PS: If you want to know more about my physics studies, click here.
Sources
- Unterschätzte Gefahr im Frühling: Der Sonnenbrand!, access: 27.09.2023
- Warum steht die Sonne unterschiedlich hoch am Himmel?, access: 27.09.2023
- UV-Strahlung, access: 27.09.2023
- Forscher klären Mechanismus des Sonnenbrands, access: 27.09.2023
- Doppelspaltexperiment, access: 27.09.2023
- Äußerer lichtelektrischer Effekt, access: 27.09.2023
- Sonnenstrahlung, access: 27.09.2023
- UV-Schutz im Auto?, access: 27.09.2023